RESEARCH
Development of Biomaterials for Intra-arterial Treatment of Cancer
Microspheres for Intra-arterial Radiotherapy or Hyperthermia
Generally, an organ diseased with cancer is resected by surgical operation. However, the functions of the resected organ are often not recovered. Therefore, it is obviously desirable to develop a treatment for cancer that can destroy only the cancerous cells, so that normal tissue can regenerate after treatment. Radiotherapy is one treatment that shows such potential; however, irradiation is performed externally in most cases. Consequently, insufficient doses of radiation are often received by the cancer-especially deep-seated cancers-and the irradiation can also cause severe damage to healthy tissues. Radioactive microspheres 20-30 µm in diameter are useful for the radiotherapy of cancers, especially for tumors located deep inside the body. These spheres are entrapped in the capillary bed of the tumors when they are implanted through blood vessels, and can irradiate cancers locally (Fig. 1).
Cancer cells generally perish at around 43 degreeC because their oxygen supply via the blood vessels is not sufficient, whereas normal cells are not damaged at even higher temperatures. In addition, tumors are more easily heated than the surrounding normal tissues, since the blood vessels and nervous systems are poorly developed in the tumor. Therefore, hyperthermia is expected to be a very useful treatment for cancer, with few side effects. Various techniques for heating tumors, such as treatments with hot water, infrared rays, ultrasound, and microwaves have been attempted. However, deep-seated tumors cannot be heated effectively and locally by using these techniques. Ferrimagnetic microspheres 20-30 µm in diameter are useful as thermoseeds for inducing hyperthermia in deep-seated cancers (Fig. 1). These spheres are entrapped in the capillary bed of the tumors when they are implanted through blood vessels, similarly to the radioactive microspheres described above, and can heat cancers locally by their hysteresis loss when placed in an alternating magnetic field. In this laboratory, we try to develop novel biomaterials for minimally invasive treatment of cancer by using various synthetic techniques (Figs. 2-6).
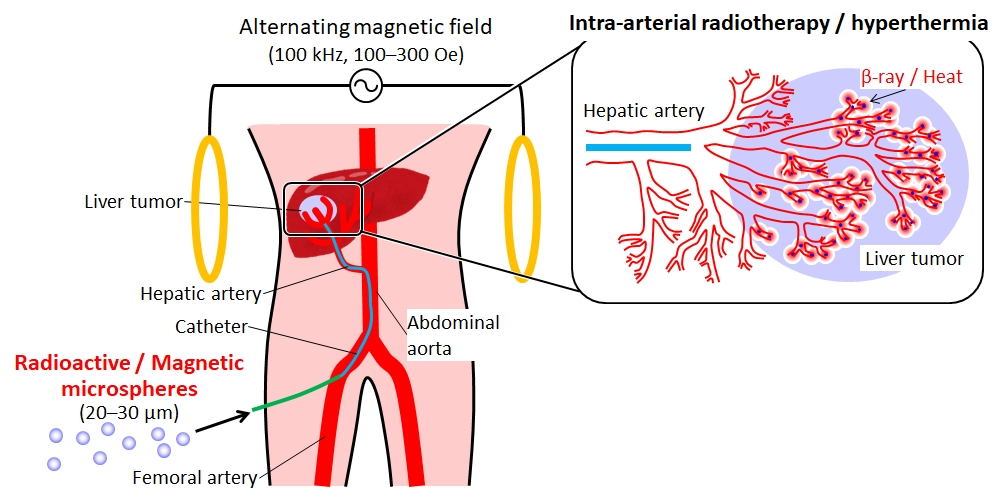
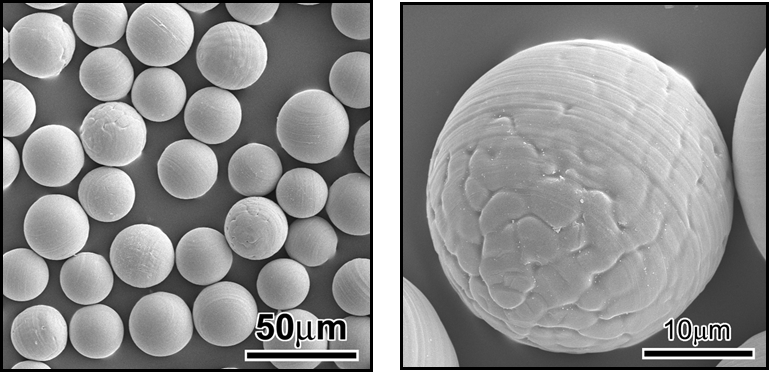
(M. Kawashita et al., Biomaterials, 24 (2003) 2955)
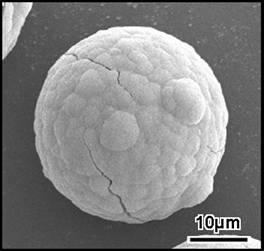
(M. Kawashita et al., Biomaterials, 26 (2005) 2231)
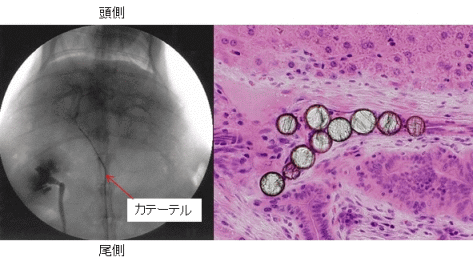
(M. Kawashita et al., Int. J. Appl. Ceram. Tech., 2 (2005) 173)
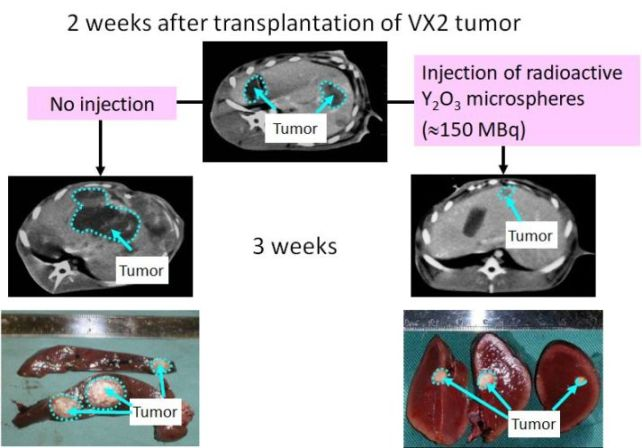
(M. Kawashita et al., Int. J. Appl. Ceram. Tech., 2 (2005) 173)
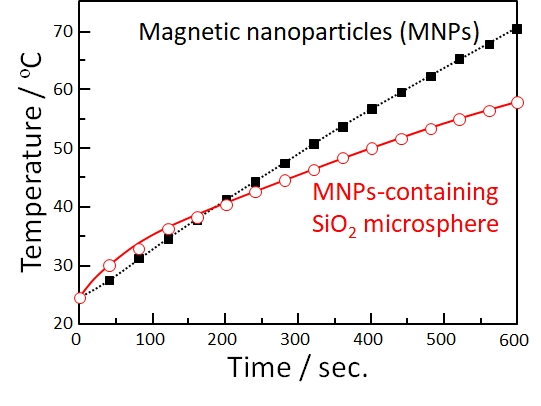
(Z. Li et al., Biomed. Mater., 5 (2010) 065010)
Development of Bioactive Materials for Bone Repair
Our body is supported by bones (Fig. 7) from the top of the head to the bottom of the feet. Important organs such as brain, heart and lung are protected from external forces by the bones. We can walk, bend and seize something, since the bone is divided into 206 parts and connected through joints.
Artificial materials implanted into bone defects are generally encapsulated by a tissue of collagen fibers to be isolated from the surrounding bone. This is a normal foreign body reaction for protecting our body from foreign materials. Because of this encapsulation, however, artificial material can not be fixed stably to the surrounding bone.
It has been found from 1970 that some ceramics can bond to living bone without fibrous tissues. They are called "bioactive" ceramics and clinically used as improtant bone substitutes. however, in a clinical field, novel bioactive materials showing excellent bone-bonding ability are required. In this laboratory, we try to develop novel bioactive materials by using various synthetic techniques (Figs. 8 and 9).
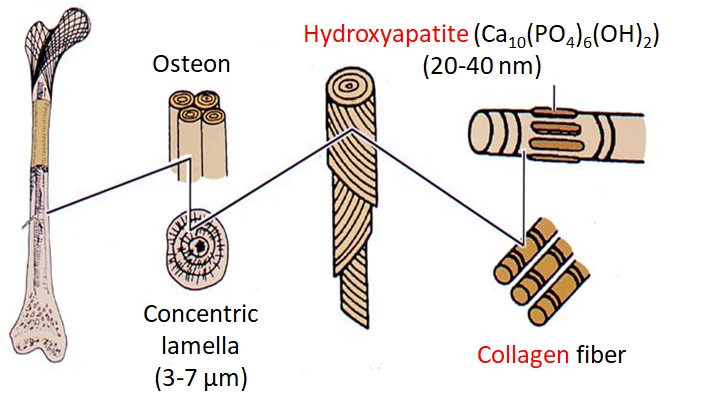
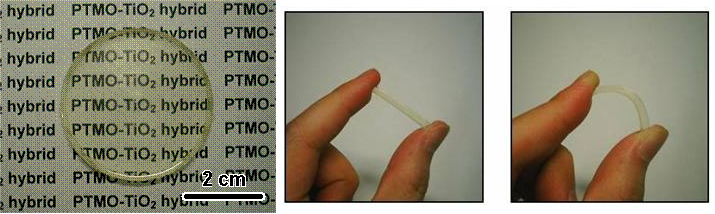
(M. Kamitakahara et al., Biomaterials, 24 (2003) 1357)
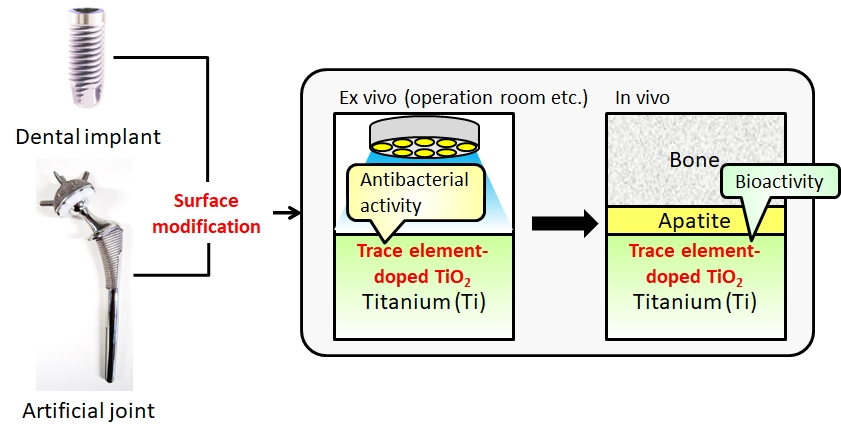
(M. Kawashita et al., Colloids Surf. B, 145 (2016) 285)
Study on Osteoconductive Mechanism of Hydroxyapatite
Hydroxyapatite (HAp) is widely used as an artificial bone or a coating material for metallic biomaterials because it bonds to living bone (shows osteoconductivity). However, the details of the expression mechanism of its osteoconductivity have not been clarified yet.
In our laboratory, we are focusing on the initial adsorption of serum proteins such as albumin, fibronectin (Fn) and laminin (Ln) on HAp and hypothesize that some serum protein which specifically adsorbs on HAp causes the osteoconductivity of HAp. So far, we found that
- Fn adsorbs more on non-osteoconductive alumina (Al2O3) than on HAp (Fig. 10), but Ln does slightly more on Al2O3 than HAp, and the crystal orientation of HAp affects the adsorption of Fn and Ln. (M. Hasegawa et al., Biomed. Mater., 11 (2016) 045006; H. Fujita et al., ACS Biomater. Sci. Eng., 2 (2016) 1162)
- Fn and/or Ln might specifically adsorb on HAp and contribute the expression of osteoconductivity of HAp because Fn-adsorbed HAp enhances the attachment and spreading of osteoblast-like cells (MC3T3-E1 cells) than Fn-adsorbed Al2O3 (Fig. 11). (M. Kawashita et al., Mater. Sci. Eng. C, 69 (2016) 1268)
- According to proteome analysis of serum proteins that adsorbs on HAp and Al2O3, 68 proteins adsorb on only HAp and 14 proteins adsorb more on HAp than Al2O3, suggesting that some proteins inducing the osteoconductivity of HAp are involved in these 82 (=68+14) proteins. (39th Annual Meeting of Japanese Society for Biomaterials, 21 November, 2017)
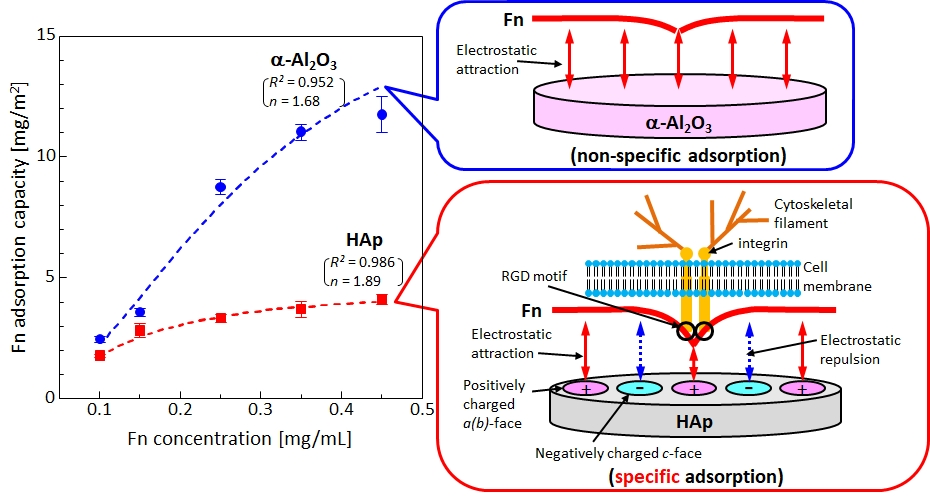
(M. Hasegawa et al., Biomed. Mater., 11 (2016) 045006)
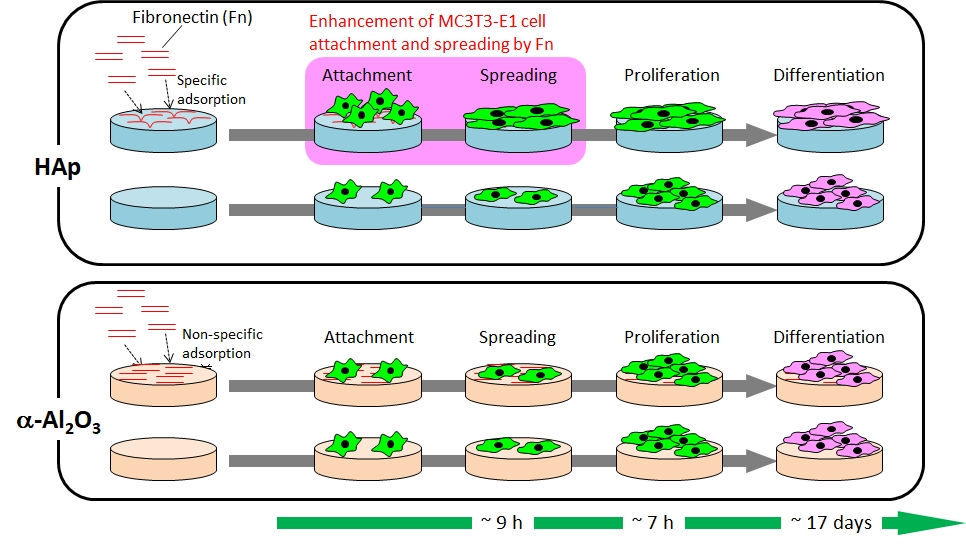